Serviços Personalizados
Journal
Artigo
Indicadores
-
Citado por SciELO
-
Acessos
Links relacionados
-
Similares em SciELO
Compartilhar
Portugaliae Electrochimica Acta
versão impressa ISSN 0872-1904
Port. Electrochim. Acta vol.34 no.3 Coimbra maio 2016
https://doi.org/10.4152/pea.201603197
Corrosion Inhibition of 1,4-di (1-vinyl-3-methylimidazolium) Benzene Dibromide on Mild Steel in HCl Solution
Ayssar Nahle* , Reem Saeed Al-tuniji , Ideisan Abu-Abdoun and Ibrahim Abdel-Rahman
Department of Chemistry, College of Sciences, University of Sharjah, Sharjah, P.O.Box: 27272, United Arab Emirates
Abstract
The inhibition and the effect of temperature and concentration of 1,4-di(1-vinyl-3methylimidazolium) benzene dibromide (VMIBB) on the corrosion of mild steel in 1.0 M HCl solution was investigated by electrochemical polarization and weight loss experiments at temperatures ranging from 303 to 343 K. The studied inhibitor concentrations ranged between 1.0×10-6 M and 1.0×10-2 M. The percentage inhibition increased with the increase of the inhibitor's concentration. The percentage inhibition has reached about 93 % at the concentration of 1×10-2 M and 303 K. On the other hand, the percentage inhibition decreased with the increase of temperature. Using the Langmuir adsorption isotherm, the thermodynamic parameters for the adsorption of this inhibitor on the metal surface were calculated. 1,4-di(1-vinyl-3-methylimidazolium) benzene dibromide was found to be a potential corrosion inhibitor, since it contained not only nitrogen, but also three aromatic systems, double bond, and it is a large molecule which has a big surface with high malar surface coverage.
Keywords: Corrosion, inhibitor, 1,4-di(1-vinyl-3-methylimidazolium) benzene dibromide, Langmuir adsorption isotherm.
Introduction
In many civil services and industries, corrosion of metals constitutes a serious economic problem. Corrosion leads to an increase in running costs, a decrease in plant efficiency, and affects the product quality. In order to protect the metal from corrosion, inhibitors are used especially when the metal is in contact with aggressive solutions, such as during acid pickling. Prior to coating by electroplating, galvanizing, or painting techniques, the metal has to go through a treatment which requires the removal of scale and salts from steel surfaces, tanks, and pipelines. In order to prevent an extensive dissolution of the underlying metal, the acid must be treated by the addition of organic inhibitors which will adsorb onto the metal/solution interface by displacing water molecules on the surface and forming a compact barrier film.
Most organic corrosion inhibitors consist of organic compounds containing polar groups by which the molecule can strongly adsorb onto the metal surface [1, 2]. The presence of the inhibitor on the metal surface decreases the reaction rate at the metal/solution interface, as it acts as a catalytic poison without being involved in the reaction. Such inhibitors contain organic N, P, S, and OH groups. Corrosion inhibition of mild steel in acidic solutions has been widely investigated. Various nitrogen-containing compounds have been used as corrosion inhibitors by several authors. These inhibitors include quaternary ammonium salts [3-10], polyamino-benzoquinone polymers [11], azoles [12], substituted aniline-N-salicylidenes [13], amides [14], heterocyclic compounds [15, 16], and cationic surfactants [17, 18]. Sulfur-containing inhibitors were also investigated by other authors [19-24]. The effect of ions addition, such as iodide [26], and chloride [27] on the inhibition efficiency of some organic compounds were studied by others. The structural effect of organic compounds as corrosion inhibitors was also studied [28, 29]. In all these studies, the nitrogen atom(s) in the compounds showed to be able to adsorb very well onto the metal surface, and form a protective layer, which, in its turn, increased the corrosion inhibition with the increase in the concentration of the inhibitor, in some cases reaching 99% inhibition [30].
No studies have been found on 1,4-di(1-vinyl-3-methylimidazolium) benzene dibromide (VMIBB) (Scheme 1) as a corrosion inhibitor for mild steel in 1.0 M HCl solution.
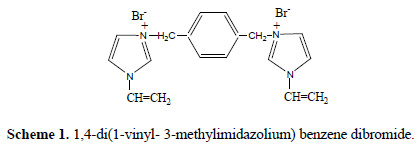
In our studies, mild steel was chosen, because high temperature aggressive acids are widely used in industries in connection to mild and low alloy steels. Because of their environmentally friendly characteristics, in addition to their unique properties, imidazolium salts are expected to be good corrosion inhibitors [31-33].
Experimental details
Synthesis of 1,4-di(1-vinyl-3-methylimidazolium) benzene dibromide
1,4-di(1-vinyl-3-methylimidazolium) benzene dibromide salt (3) was prepared by refluxing two molar equivalent from vinylimidazole (1) with one molar equivalent from 1,4-dibromethybenzene (2) in acetone, as shown in the procedure below (Scheme 2):
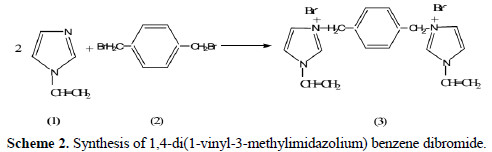
A mixture of 6.20 grams (0.066 mol) of vinylimidazole (1), and 8.72 grams of 1,4 dibromethylbenzene (0.033 mol) (2), in 500 mL carbon tetrachloride (CCl4) was refluxed for 6 hours. After cooling to room temperature, a crystalline off-white precipitate (3) was collected by filtration, then washed with excess diethyl ether and dried. Dissolution in small amount of chloroform and addition of excess diethyl ether gave the pure salt (3) in 80 percent yield with a melting point between 133 and 136 °C.
Electrochemistry
Electrode preparation
A 5 mm diameter piece cut from a mild steel rod (IS 226 containing 0.18 % C, 0.6 % Mn, and 0.35 % Si) supplied by ''Reliable Steel Traders'', Sharjah, UAE, formed the working electrode; and was mounted, using Araldite epoxy resin, in a glass tube that fits in the electrochemical cell. Prior to each experiment, the working mild steel electrode was abraded using a series of carborundum papers starting with 600 grades and ending with 1200 grades. The electrode surface was then polished with 0.3-μm alumina on cloth, washed with deionized distilled water, and rinsed with pure ethanol, before being transferred to the electrochemical cell that contained deaerated fresh electrolyte.
Instrumentation
Fig. 1 shows the electrochemical cell which consisted of a mild steel working electrode (WE), a saturated calomel electrode (SCE) as a reference electrode (RE), and platinum gauze counter electrode (CE).
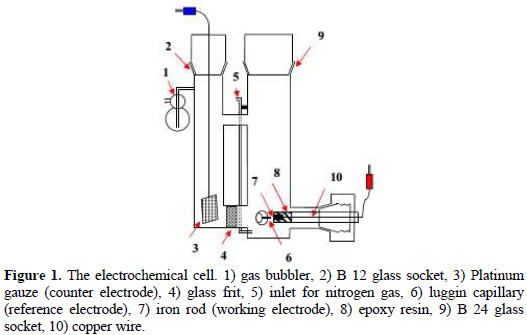
Prior to each experiment, nitrogen was bubbled to deaerate the electrolyte. This cell was designed in a way that the nitrogen was allowed to escape into the solution, precluding its collection at the electrode surface.
The counter-electrode compartment was separated from the working-electrode compartment with a glass frit in order to protect the working electrode from any substance that may be produced at the counter electrode during the electrochemical reactions. The following electrochemical instrument was used: A PC controlled AUTOLAB PGSTAT128N Modular Potentiostat (electrochemical workstation) (supplied from Metrohm) capable of driving currents up to ± 800 mA with an output potential across the cell of up to ± 10 V.
Measuring procedure
Electrochemical corrosion measurements (Tafel plots) were carried out on the mild steel electrode, prepared as described before, in 1.0 M HCl and in 1.0 M HCl containing various concentrations of the prepared VMIBB inhibitor. The concentration of the inhibitor ranged from 1×10-6 M to 1×10-2 M.
After filling the cell with 60 mL of the electrolyte, the solution was deaerated with nitrogen gas, and the WE equilibrium potential was monitored and recorded vs. SCE, until it reached a steady state. The electrode potential was then scanned between overpotentials of -100 mV to +100 mV vs. SCE at a sweep rate of 1 mV.s-1.
Results and discussions
The anodic and cathodic polarization curves (Tafel plot) of the mild steel electrode in deaerated 1.0 M HCl solution, with and without the addition of various concentrations of 1,4-di(1-vinyl-3-methylimidazolium) benzene dibromide (VMIBB), are shown in Fig. 2.
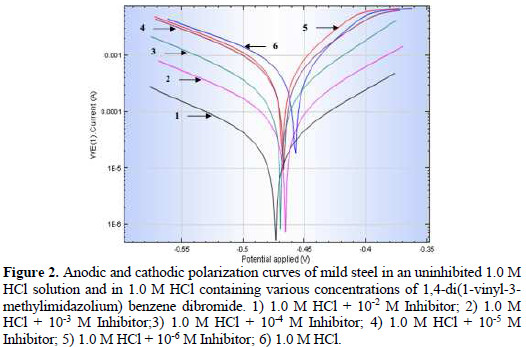
Both the anodic and cathodic branches of the curve were affected by the presence of the inhibitor. The corrosion current decreased with the increase of the concentration of VMIBB inhibitor, as shown in Table 1.
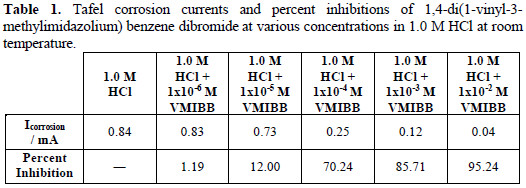
In the absence of the inhibitor (in 1.0 M HCl), the corrosion current was found to be 0.84 mA, which decreased sharply to 0.038 mA at 1×10-2 M of VMIBB inhibitor in 1.0 M HCl (reaching about 95% inhibition) (Table 1).
The values of the percentage inhibition of VMIBB at various concentrations in 1.0 M HCl were calculated according to the following equation and the results and are shown in Table 1:

where: ( Icorr )Uninh is the corrosion current in the uninhibited solution, and ( Icorr )Inh is the corrosion current in inhibited solution.
Table 1 shows that the percentage inhibition increases gradually from about 1% (with 1×10-6 M inhibitor) to reach about 95% with 1×10-2 M inhibitor.
Effect of temperature
Specimen preparation
Rectangular specimens (1 cm × 2.3 cm × 0.3 cm) cut from a large sheet of 3 mm thick mild steel (IS 226 containing 0.18 % C, 0.6 % Mn, and 0.35 % Si), supplied by ''Reliable Steel Traders'', Sharjah, UAE; were used for weight loss measurements. A 2-mm diameter hole was drilled close to the upper edge of the specimen, and served to be hooked with a glass rod for immersion purposes. Prior to each experiment, the specimens were polished with 600 grade emery paper, rinsed with distilled water, degreased with acetone, dried, and finally weighed precisely on an accurate analytical balance.
Instrumentation
For the weight-loss measurements, a 250 mL round bottom flask fitted with a reflux condenser and long glass rod, which served to hook and immerse the specimen and in turn immersed in a thermally controlled water bath, was used.
Measuring procedure
The flask was filled with 100 mL of 1.0 M HCl solution with and without (VMIBB) of various concentrations, then placed in water bath. As soon as the required working temperature was reached, the mild steel sample was immersed in the solution, and left there for exactly six hours, after which the sample was removed, rinsed with distilled deionized water, degreased with acetone, dried, and finally weighed precisely on an accurate analytical balance. This procedure was repeated with all the samples, with a variety of inhibitor concentrations ranging from 1×10-6 M up to 1×10-2 M; and at temperatures ranging from 303 to 343 K. These experiments were repealed for reproducibility, and the average values were reported.
Results and discussions
Effect of temperature
Weight loss corrosion tests were carried out on the mild steel in 1.0 M HCl in the absence and presence of (VMIBB) over a period of 6 hours. Table 2 represents the corrosion rates [mg.cm-2.h-1], and the percentage efficiencies [%] for the studied inhibitor with concentrations varying from 1×10-6 M to 1×10-2 M at 303, 313, 323, 333, and 343 K, respectively.
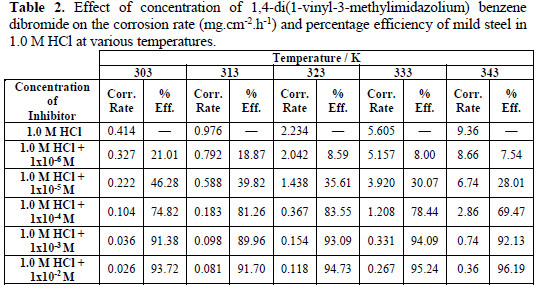
The percentage efficiency was calculated according to the following expression:

where CRuninh and CRinh are the corrosion rate without and with inhibitor, respectively.
Fig. 3 and Fig. 4 show the plots of the corrosion rate of (VMIBB) as a function of concentration at 303, 313, 323, 333, and 343 K.
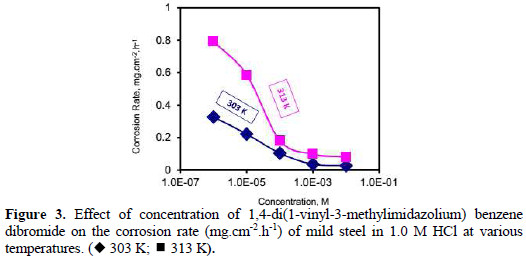
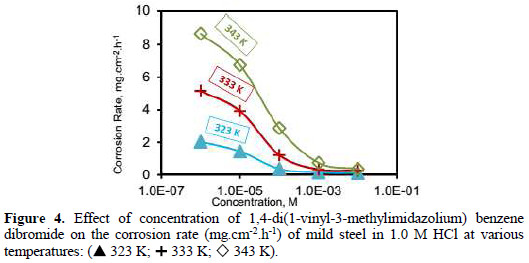
At 303 K (Fig. 3) the corrosion rate dropped from 0.414 mg.cm-2.h-1 (1.0 M HCl in the absence of the inhibitor) to 0.327 mg.cm-2.h-1, when 1×10-6 M of (VMIBB) was present in the 1.0 M HCl. This corrosion rate continued to decrease slightly to reach 0.222 mg.cm-2.h-1 (46.3 % inhibition) at a concentration of 1×10-5 M, followed by a further slow decrease to reach 0.104 mg.cm-2.h-1, when the inhibitor's concentration was 1×10-4 M; and kept on decreasing to reach 0.026 mg.cm-2.h-1 (93.7 % inhibition) at a concentration of 1×10-2 M. At 313 K (Fig. 3), the curve showed a steep decrease in corrosion rate between 1×10-6 M and 1×10-4 M, followed by a slow decrease to form a plateau between 1×10-3 M and 1×10-2 M, where the inhibition reached about 92%.
At 323 K (Fig. 4), a slow decrease in the corrosion current occurred between 1×10-6 M and 1×10-4 M and remained steady at higher concentrations to reach 0.118 mg.cm-2.h-1 at 1×10-2 M.
At 333 and 343 K (Fig. 5) the presence of the (VMIBB) inhibitor caused a sharp decrease in the corrosion rate between 1×10-6 M and 1×10-4 M, followed by a slow decrease at higher concentrations, reaching 0.267 mg.cm-2.h-1 (95.24% inhibition), and 0.36 mg.cm-2.h-1 (96.19% inhibition) for 1×10-2 M inhibitor employed at 333 K and 343 K, respectively.
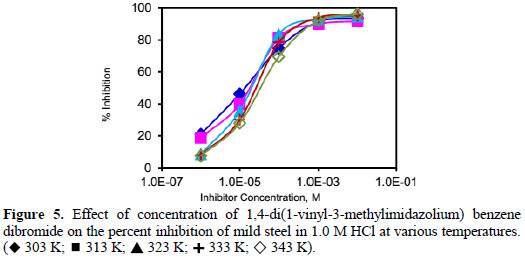
The data obtained from the weight loss measurements were plotted in accordance to Arrhenius equation:

where Ea is the activation energy [kcal.mol-1], R is the gas constant [kcal.mol-1], T is the temperature [K], and const. = constant.
The Arrhenius plots of the corrosion of mild steel in 1.0 M HCl solution (Ln corrosion rate as a function of 1/T) (Table 3), with and without the presence of (VMIBB) at concentrations ranging from 1×10-6 M to 1×10-2 M, are presented in Fig. 6.
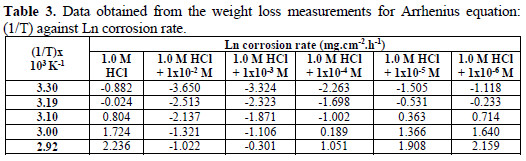
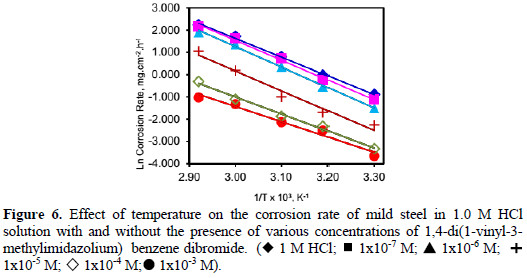
From Fig. 6, the slope ( -Ea/R ) of each individual line was determined and used to calculate the activation energy according to equation 2, and taking R=1.987×10-3 kcal.mol-1 (Table 4).
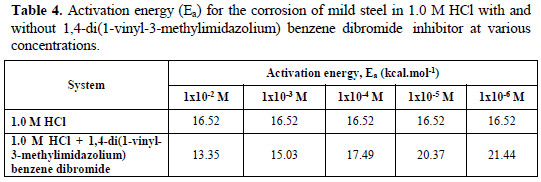
In the presence of high inhibitor concentrations (1×10-2 M and 1×10-3 M), caused a decrease in the activation energy (initially 16.52 kcal.mol-1), which could be attributed to chemisorption due to charge sharing or transfer from the organic inhibitor to the metal surface to form coordinate covalent bonds, while an increase in the activation energy, occurred at low concentrations of the inhibitor (1×10-4 M to 1×10-6 M), is due to physisorption [34].
The surface coverage of various concentrations of (VMIBB) (ranging from 1×10-6 M to 1×10-2 M) on mild steel surface as a function of temperature are shown in Table 5.
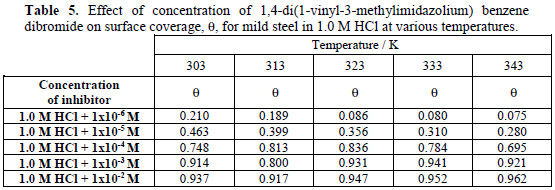
The dependence of the fraction Cinh/θ as a function of Cinh of (VMIBB) is shown in Fig. 7.
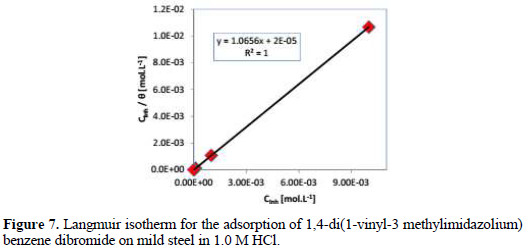
The obtained plot of the inhibitor is almost linear, with a correlation coefficient about 1.00.
The plot of Cinh/θ versus Cinh yielded a straight line with nearly unit slope and the best fits are obtained with Langmuir adsorption isotherm, as presented in Fig. 7. It was found that the linear correlation coefficient is very close to 1.000, which clearly proves that the adsorption of (VMIBB) from 1.0 M hydrochloric acid solutions on mild steel obeys the Langmuir adsorption isotherm, which is described by the following equation:

where θ is the degree of coverage, Kads is the equilibrium constant for the adsorption reaction, and Cinh is the concentration of the inhibitor.
From the intercept of the straight line Cinh/θ = f(Cinh), kads value can be calculated.
The equilibrium constant for the adsorption reaction, K, is related to the standard free energy of adsorption via the following equation:

where Keq is the equilibrium constant for the adsorption reaction, ΔG is the standard free energy [kcal.mol-1], R is the gas constant [kcal.mol-1], and T is the absolute temperature [K].
Rearrangement of (Equation 5) leads to Equation 9.




The standard free energy change of adsorption of the inhibitor, ΔG0, can be calculated from the results in Figure 7 used to calculate the equilibrium constant, Keq, and Equation 9 at various temperatures (303 to 343 K).
The standard enthalpy change of adsorption, ΔH0, for the inhibitor can be calculated from the following equation:

The entropy, ΔS0, can be calculated at various temperatures for the inhibitor using Equation 11:

Tables 6, 7 and 8 show the thermodynamic data obtained in the presence of the inhibitor at 1×10-2 M.
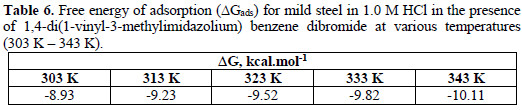
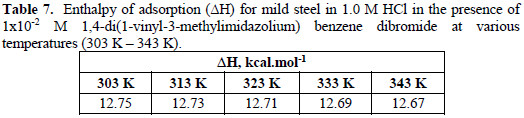
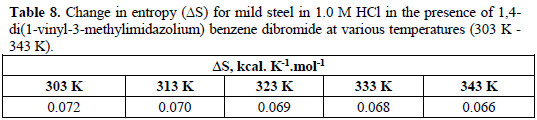
These thermodynamic quantities represent the algebraic sum of the values for adsorption and desorption. The negative value of ΔG0 (Table 6) indicates the spontaneous adsorption of inhibitor on the surface of the mild steel. The calculated standard free energy, ΔG0, varied from -12.39 kcal.mol-1 at 303 K to -13.26 kcal.mol-1 at 343 K.
The standard enthalpy change of adsorption for the inhibitor, ΔH0, is shown in Table 7. The positive values of the ΔH are due to the fact that, in order for the inhibitor to get adsorbed on the metal substrate (adsorption process is exothermic, negative enthalpy), water molecules which are already adsorbed on the surface have to get desorbed into the solution (desorption process is endothermic, positive enthalpy). Since an enormous number of water molecules were initially adsorbed on the metal surface, a large amount of energy is provided to achieve this endothermic process; on the other hand, a small number of inhibitor molecules will replace the water at the metal surface, which, in its turn, will release a small amount of energy during this adsorption process.
The adsorption process is exothermic and associated with a decrease in entropy (ΔS) of solute, while the opposite is true for the solvent. The gain in entropy which accompanies the substitutional adsorption process is attributed to the increase in the solvent entropy (Table 8). This agrees with the general suggestion that the values of ΔG0 increase with the increase of inhibition efficiency [22, 35], as the adsorption of the organic compound is accompanied by desorption of water molecules off the surface.
The high inhibition efficiency may be attributed to the preferred flat orientation of this compound on the metal surface. The interaction occurs between the delocalized π-electrons of the three aromatic rings, the two double bonds (- C = C -), and the lone pair of electrons on N atoms with the positively charged metal surface. In addition, the two double bonds (- C = C -) play a major role in the stabilization of both sides of the inhibitor on the surface of the metal.
Conclusions
1,4-di(1-vinyl-3-methylimidazolium) benzene dibromide was found to be a very efficient inhibitor for mild steel in 1.0 M HCl solution, reaching about 94 per cent at a considerably low concentration (1×10-2 M) at 303 K. The results obtained from electrochemical and weight loss measurements are in mutual agreement.
(VMIBB) is a potential corrosion inhibitor, since it contains not only a nitrogen atom, but also three phenyl rings. It was apparent from the molecular structure that this compound would be adsorbed onto the metal surface through the lone pair of electrons of nitrogen and p-electrons of the three aromatic rings, as well as the two double bonds that are attached to the imidazole rings. The percentage inhibition in the presence of this inhibitor decreased with the increase of temperature at low concentrations 1×10-6 M to 1×10-4 M, which indicated that physical adsorption was the predominant inhibition mechanism. However, the percentage inhibition in the presence of this inhibitor was not affected with temperature at high concentrations (1×10-3 M and 1×10-2 M), which indicates a chemical adsorption on the surface of the metal.
References
1. Damaskin B B, Pietrii O A, Batrokov W W. Adsorpcja organiczeskich sojedinienij na electrodach. Moskva; 1968. [ Links ]
2. Okamato G, Nagayama M, Kato J, et al. Corrosion Sci. 1962;2:21. [ Links ]
3. Beloglazov S M, Dzhafarov Z I, Polyakov V N, et al. Protection Metals USSR. 1991;27:810. [ Links ]
4. Fokin A V, Pospelov M V, Levichev A N. Protection Metals USSR. 1983;19:242. [ Links ]
5. Nahle A. Corrosion Prevent Control. 1997;44:99. [ Links ]
6. Nahle A. Corrosion Prevent Control. 1998;45:124. [ Links ]
7. Nahle A. Bull Electrochem. 2002;18:105. [ Links ]
8. Nahle A, Walsh F C. Corrosion Prevent Control. 1995;42:30. [ Links ]
9. Savithri B V, Mayanna S M. Ind J Chem Technol. 1996;3:256. [ Links ]
10. Vasudevant T, Muralidharan S, Alwarappan S, et al. Corrosion Sci. 1995;37:1235. [ Links ]
11. Muralidharan S, Phani K L N, Pitchumani S, et al. J Electrochem Soc. 1995;142:1478. [ Links ]
12. Popova A. Corrosion Sci. 2007;49:2144. [ Links ]
13. Talati J D, Desai M N, Shah N K. Mater Chem Phys. 2005;93:54. [ Links ]
14. Tuken T, Yazici B, Erbil M. Turk J Chem. 2002;26:735. [ Links ]
15. Fattah A A, Mabrouk E M, Abd El-Gulil RM, et al. Bull Soc Chimique France. 1991;1:48. [ Links ]
16. Granese S L, Rosales B M, Oviedo C, et al. Corrosion Sci. 1992;33:1439. [ Links ]
17. Al-Lohedan H A, Khamis E, Issa Z A. Adsorption Sci Technol. 1996;13:137. [ Links ]
18. Qiu L G, Xie A J, Shen Y H. Mater Chem Phys. 2005;91:269. [ Links ]
19. Ateya B C, El-Anadouli B E, El-Nizamy F M. Corrosion Sci. 2005;24:497. [ Links ]
20. Nahle A. Bull Electrochem. 2001;17: 221. [ Links ]
21. Nahle A. Bull Electrochem. 2005;21:275. [ Links ]
22. Fouda A S, Mousa M N, Taha F I, et al. Corrosion Sci. 1986;26:719. [ Links ]
23. Raicheva S N, Aleksiev B V, Sokolova E I. Corrosion Sci. 1993;34:343. [ Links ]
24. Sanad S H, Ismail A A, El-Meligi A A. Bull Electrochem. 1995;11:462. [ Links ]
25. Zucchi F, Trabanelli G, Brunoro G. Corrosion Sci. 1992;33:1135. [ Links ]
26. Huang Y L, Goa C N, Lu M, et al. Corrosion. 1993;49:644. [ Links ]
27. Yamaguchi M, Nishihara H. Corrosion Sci. 1994;36:1133. [ Links ]
28. Kobayashi K, Shimizu K, Iida M. Corrosion Sci. 1993;35:1431. [ Links ]
29. Skryler L D, Streltsova E A, Skryleva T L. Protection Metals USSR. 1991;27:755. [ Links ]
30. Nahle A, Abu-Abdoun I, Abdel-Rahman I. Anti-Corrosion Methods Mater. 2007;54:244. [ Links ]
31. Popova A, Christov M, Vasilev A. Corrosion Sci. 2015;94:70. [ Links ]
32. Nahle A, Abu-Abdoun I, Abdel-Rahman I. Anti-Corrosion Methods Mater. 2008;55:217. [ Links ]
33. Zhao H. Chem Eng Comm. 2006;193:1660. [ Links ]
34. Popova A, Christov M, Deligeorgiev T. Corrosion. 2003;59:756. [ Links ]
35. Fouda A S, El-Aal A A, Kandil A B. Anti-Corros Methods Mater. 2005;52:96. [ Links ]
*Corresponding author. E-mail address: anahle@sharjah.ac.ae
Received 10 April 2016; accepted 28 April 2016