Serviços Personalizados
Journal
Artigo
Indicadores
-
Citado por SciELO
-
Acessos
Links relacionados
-
Similares em SciELO
Compartilhar
Portugaliae Electrochimica Acta
versão impressa ISSN 0872-1904
Port. Electrochim. Acta vol.29 no.3 Coimbra jul. 2011
High Temperature Interaction of NiO with Sodium Sulphate in SO2 Environment at 1100 and 1200 K
S.K. Hasan*,1 and M. Mobin2
1Dept. of Applied Chemistry, Institute of Technology for Women, Gorakhpur, India 2Dept. of Applied Chemistry, Aligarh Muslim University, Aligarh, India
Abstract
The present paper contains the results relative to the reaction of NiO with Na2SO4 in presence of SO2 (g) at 1100 and 1200 K. Nickel oxide (NiO) is produced by the oxidation of metal alloys in many industrial operations. The oxidation of metal and metal oxides in presence of molten salts and gas environment at high temperature is often called as hot corrosion. In this investigation the studies have been carried out taking into consideration the influence of SO2 partial pressure. The thermo-gravimetric studies have been carried out as a function of Na2SO4 concentration in the mixture. The reaction products identified by XRD analysis and surface morphologies of the products were discussed on the basis of metallographic and scanning electron microscopic (SEM) studies. The pH of the aqueous solutions of the reaction products were measured to observe the changes in the system with varying the Na2SO4 concentration. A quantitative estimation of soluble metal ions has also been carried out with the help of an atomic absorption spectrophotometer. The possibility of chemical reaction is established by thermodynamic calculations of free energies of the reaction products.
Keywords: high temperature, hot corrosion, metal oxide, sodium sulfate, XRD, SEM, and NiO.
Introduction
The high temperature oxidation resistance of alloys depends on the formation of a protective and adherent oxide layer on the metal surface. The oxide scale must be dense, homogenous, well adhered and stable as possible as to constitute a good diffusion barrier. NiO is a protective corrosion resistant coating over a base metal. The oxide does not alter the dimension, physical or chemical properties of the surface treated. The coating does not chip or peel off and is primarily used for decorative purposes. The nickel oxide conversion coating can be used as an impregnate for metalized coatings, for use in chlorine-containing environments such as the metal surfaces of refuse-burning boilers. These oxides are generally formed by oxidation at high temperature of the corresponding elements initially present in the alloy. A wide variety of electrolytic deposits is known to cause hot corrosion, but Na2SO4 has received greatest attention due to its involvement in actual engineering systems. Because of its high thermodynamic stability in the mutual presence of sodium and sulphur impurities in an oxidizing gas, Na2SO4 is often found to be the dominant salt in the deposit. The sputtered nickel deposit is consistently more protective against corrosion than an electrolytic deposit of the same thickness [1]. In a recent study, corrosion of high-purity nickel with deposits of sodium sulfate has been studied at 900 °C in O2 and SO2 at gas pressures ranging from 700 to 0.5 Torr [2]. A considerable work has been reported on the influence of noble metal addition on hot corrosion behaviour of high temperature alloys. Goebel et al. [3] examined hot corrosion of Ni-based alloys containing Cr, Al, Mo, W and V additions, reviewed and discussed excellently by Stringer [4] and Rapp et al. [5].
One of the factors that affect the oxidation resistance of protective oxide scales is their solubility in molten Na2SO4. A review of the literature on hot corrosion reveals that only the work carried out by Rapp and co-workers on the solubility of metal oxides in molten Na2SO4 provides some information about metal oxide-Na2SO4 reaction at high temperature [6,7]. Information regarding the reaction between a pertinent oxide and Na2SO4 and proper identification of reaction products should be useful in understanding the occurrence and importance of fluxing reactions, and thus in the interpretation of hot corrosion mechanism and in the development of new protective materials. The dissolution behaviour of metal oxide is important in explaining the electro-chemical mechanism of hot corrosion in which soluble metal species are involved. The results of the study of high temperature interaction of some transition and non-transition metal oxides with Na2SO4 in oxygen have recently been published [8-10]; where the reaction was performed in pure O2(g) atmosphere the influence of SO2/SO3 partial pressure on the reaction product was not considered.
This paper contains the results of the studies concerning with the reaction of NiO and Na2SO4 in flowing SO2(g) in the temperature range of 1100 and 1200 K where hot corrosion reactions usually show optimization. In the present investigation, studies have been carried out taking into consideration the effect of SO2 gas partial pressure. The oxide selected for the studies is present on the scales formed on Na2SO4 during the initiation stage of hot corrosion attack and reacts with Na2SO4 during the propagation stage by fluxing reaction.
Experimental
Chemicals used
NiO analytical reagent grade BDH product and analytical grade Na2SO4 and oxides of about 80 mesh size dried in an oven at 200 °C for about 48 hours were used.
Preparation of the specimens
Dried and powdered Na2SO4 and nickel oxide were mixed separately in 1:2, 2:3, 1:1, 3:2 and 2:1 molar ratios of Na2SO4 and nickel oxide was put in a die (diameter 1.4 cm) and pressed into compact tablets using a hydraulic press (pressure: 107 kg m-2).
Oxidation studies
The kinetics of the reaction between Na2SO4 and nickel oxide was studied by monitoring weight change as a function of time at 1100 and 1200 K in a stream of SO2 gas (purity 99.9%) using a hot stage Sartorius electronic microbalance. A 20-hour oxidation run was adequate to provide reaction products at steady state, which was indicated by a negligible change in weight for a considerable period of exposure time.
To obtain a sufficient number of samples of the reaction products for a particular system, 3 silica boats, and each containing compacts of the same ratio were placed in a horizontal furnace for oxidation under almost identical conditions. After completion of the oxidation, the compacts were taken out and quenched in air and weighed. Out of three samples, one was mounted for morphological studies (XRD, metallographic and SEM), the second for qualitative chemical analysis, conductivity and pH determination and the third was retained for quantitative determination of soluble metal species.
X-ray diffraction studies
The X-ray diffraction studies were carried out using a Philips X-ray diffractometer (Model PW 1730 X-ray generator with PW 1710 microprocessor and KSR 43 printer) with Cu Kα target and appropriate filter.
Metallographic studies
Metallographic studies were carried out on a computerized Leitz photometallurgical microscope (Metallux 2). The reaction products present in the form of compacts were mounted in paper moulds using Araldite as a cold setting resin. The mounted specimens were ground on 600 grit SiC paper followed by polishing with 6μ diamond paste using kerosene as the lapping liquid. Appropriate etchants were used to identify the different phases in the microstructures. Table 1 lists the different etchants used in the metallographic studies.
Table 1. Etchants used in metallographic studies.

Scanning electron microscopic (SEM) studies
SEM studies were performed using a Philips 505 electron microscope. Polished specimens were coated with colloidal gold emulsion and their structures were examined through the microscope. The desired portions of the microstructure of the specimen were photographed at various magnifications.
pH measurements
pH of aqueous solutions of the reaction products were measured using an Elico CM 180 pH meter. Compacts of the reaction products were dissolved in demineralized water, and the pH of the aqueous solutions were measured.
Estimation of the soluble metals
An atomic absorption spectrophotometer (Model GBC 902) was used to determine the concentration of the metals in the aqueous solutions of the reaction products for Na2SO4-nickel oxide systems. The compacts of the reaction products were weighed and dissolved in fixed volume of demineralized water. The mixture was heated near to boiling followed by filtration through Whatman filter paper. The filtrate, with appropriate dilution, was used to determine the concentration of metals in the solution.
Results
Thermo gravimetric studies
Reaction kinetics
Fig. 1 represents the total weight change (in percent) versus time plots obtained during the reaction of 1:1 mixture of NiO and Na2SO4 at 1100 and 1200 K. The salient features of the results from reaction kinetics for the NiO-Na2SO4 system are given as follows.
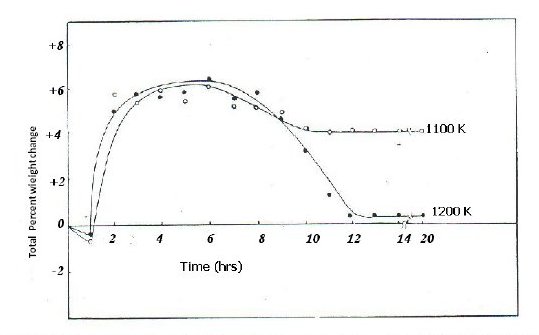
Figure 1. Plots of total percent weight change vs. time for NiO-Na2SO4 system (molar ration 1:1) at 1100 and 1200 K.
At 1100 and 1200K, after a small weight loss during the initial stages of reaction, there was rapid weight gain up to 6 hours followed by a decrease in weight gain values up to 10 hours with no further changes in weight gain on exposure to SO2. The net change in weight after 20 hours of heating was weight gain at 1100 and 1200 K. However, the final weight gain at 1200 K was less in comparison to the final weight gain at 1100 K.
Influence of salt
Fig. 2 shows plots of percent total weight change at steady state versus mole fraction of Na2SO4 in the mixture of Na2SO4: NiO. The total weight change represents the final reading recorded after heating for 20 hours, a mixture of Na2SO4 and metal oxide of known composition in SO2 (g) at 1100 and 1200 K. No further change in weight was observed after 20 hours. The results of the study of weight change versus mole fraction of Na2SO4 for NiO- Na2SO4 system can be generalized as follows.
Figure 2. Plots of total percent weight change vs. mole fraction of Na2SO4 for NiONa2SO4 system (molar ration 1:1) at 1100 and 1200 K.
The reaction of NiO with Na2SO4 at 1100 and 1200 K resulted in net weight gain. There was a decrease in weight gain with increasing the amount of Na2SO4 till a minimum is obtained at 0.5 or 0.6 mole fraction of Na2SO4, followed by an increase in weight gain values.
Variation in pH
The pH of aqueous solution of Na2SO4-NiO reaction product was measured at room temperature. Fig. 3 represents plots showing variation in pH of the aqueous solution of the reaction products with variation in the amount of Na2SO4 in the reaction mixture. A study of the pH vs. mole fraction of Na2SO4 plots indicated that the solutions are acidic at both the temperature, i.e., 1100 and 1200 K. The systems showed a distinct maximum at 0.5 mole fraction of Na2SO4 in the curves. There is a decrease in the acidic character of the solution with increasing Na2SO4 concentration till a maximum is obtained followed by an increase in acidic character with further increase in Na2SO4 concentration.
Figure 3. Plots of the pH of the aqueous solution of the reaction product vs. mole fraction of Na2SO4 for Na2SO4-NiO system at 1100 and 1200 K.
X-ray diffraction analysis
Table 2 lists the different constituents as identified in the reaction products of metal oxides and Na2SO4 at 1100 and 1200 K by X-ray diffraction analysis.
Table 2. Different constituents identified in the reaction product by X-ray diffraction analysis.

Metallographic and SEM studies
Fig. 4 shows some representative photomicrographs of mounted reaction products. The salient features of the results from metallographic studies for the NiO-Na2SO4 systems are generalized as follows.
Figure 4. SEM picture of the reaction products of NiO-Na2SO4 system (molar ratio 1:1), oxidized at (a) 1100 K (1000x) and (b) 1200 K (800x).
NiO-Na2SO4 system
The photomicrographs of the reaction products showed a multiphase structure. NiO or Na2SO4 appeared as whitish grey, NiS as dark grey and NaNiO2 as light grey. The crystalloids of NiS seem to be cooled from liquid state and spheriodized. They were embedded between NiO and NaNiO2 grains.
Estimation of soluble metal species
The concentration of soluble metal species in the reaction products of nickel oxide and Na2SO4 was determined as a mole fraction of Na2SO4 in the reaction mixture. Table 3 provides metal solubility data for Na2SO4-NiO systems at 1100 and 1200 K.
Table 3. Metal solubility data for Na2SO4-metal oxide systems at 1100 K and 1200 K, 12 h.
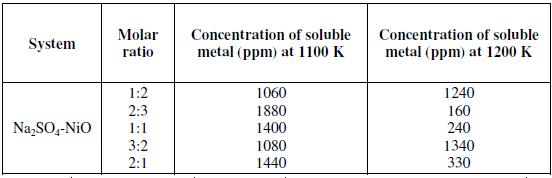
Nickel oxide showed maximum solubility at 1100 K for Na2SO4-NiO molar ratio 2:3. NiO showed maximum solubility at lower concentration of Na2SO4 (0.4 mole fraction of Na2SO4). At 1200 K the concentration of soluble NiO was observed lesser than that at 1100 K.
Discussion
The reaction of Na2SO4 with NiO at high temperatures resulted in weight gains or weight losses. The total weight change at steady state was measured. Steady state is indicated when there is no further change in weight with increasing exposure time. Considering the reaction kinetic (1:1 molar ratio), all the NiO-Na2SO4 systems showed a weight loss in very initial stages of the reaction, followed invariably by a weight gain in the systems. The initial weight loss in the system was presumably due to the thermal decomposition of salt to Na2O, and subsequent expulsion of SO2/SO3 (g). This is represented by the following reactions [7].

If the thermodynamic conditions permit then the Na2O formed may dissolve nickel oxide to give sodium nickelate. This can be represented as follows:

The sulphur oxide gases may also react with metal oxide to form metal sulphide and / or metal sulfate. This is represented by the following reactions:

The weight gain after initial weight loss in the kinetic curves for the systems was due to the formation of sodium nickel oxide and nickel sulfide and / or nickel sulfate and is represented by the reactions (3-5). The evidence for the formation of the above reaction products is inferred from the following results: (i) identification of reaction products by X-ray diffraction analysis, (ii) standard free energy calculations favorable for the proposed reactions (Table 4) and (iii) weight gain after initial weight loss in the kinetic curves for the systems. The free energy minimization calculations show that reaction (4) is possible, and reaction (5) can undergo under the experimental conditions of lower pO2 and lower basicity in the systems under study. The metallographic and SEM studies carried out on the mounted reaction products also indicated the presence of multiphase structure.
Table 4. Standard free energy of the reaction, ΔG0 at 1200 K [11,12].
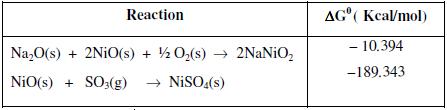
There was a sudden interruption in weight gain curves and a decrease in weight gain was observed till net weight change is weight loss. The release of SO2/SO3 (g) and the vaporization of some reaction products undoubtedly seemed to be the prime cause of the decrease in weight gain values in the kinetic curves.
Studying the total weight change as a function of mole fraction of Na2SO4 in the reaction mixture, in case of NiO-Na2SO4 system there is a decrease in the weight gain values with increasing Na2SO4 concentration till a minimum is noticed at certain mole fraction of Na2SO4; this is followed by an increase in the weight gain values with increasing Na2SO4 concentration. A decrease in weight gain values with increasing Na2SO4 concentration is due to the evaporation of sulfur oxide gases which overweighs the formation of nickel sulfate and/or nickel sulfide. When concentration increases above 0.5 or 0.6 mole fraction of Na2SO4, formation of nickel sulfate/nickel sulfide takes place, which is responsible for weight gain.
A study of pH of the aqueous solutions of the NiO-Na2SO4 reaction products indicates that solutions are acidic. An acidic aqueous solution indicates the presence of metal ions as acidic salts. The system showed a decrease in acidic character with increasing Na2SO4 concentration. After pronounced maximum at certain mole fraction of Na2SO4, again there is an increase in acidic character with further increase in Na2SO4 concentration in the reaction mixture. A decrease in acidic character of the solution is because of the formation of basic species such as nickelate. A further increase in acidic character of the solution with increase in Na2SO4 concentration is due to the precipitation of nickel oxides from basic species, which again interact with the salt to form acidic salts.
The photomicrographs of the reaction products showed the presence of multi phase structures. Each phase represents a constituent usually identified by XRD and predicted by proposed reactions. In general, an oxide phase or Na2SO4 appear as whitish grey, sulfide or sulfate as dark grey, and sodium nickel oxide as light grey.
The solubility data indicated the formation of water-soluble metal containing species in the reaction products. The metal species present in the solutions are in the form of NaNiO2, NiSO4.
Conclusions
1. High temperature reactions of NiO and Na2SO4 result initially in weight losses followed by weight gains. The weight losses are due to the thermal decomposition of Na2SO4 and subsequent expulsion of SO2/SO3 (g). The weight gains are due to formation of sodium nickel oxide (NaNiO2), nickel sulfate and/or nickel sulfide.
2. The solubility data indicate the formation of water-soluble metal containing species in the reaction products. The metal species present in the solutions are in the form of NaNiO2, NiSO4.
3. The solubility of nickel oxide was found higher at 1100 K.
4. The aqueous solution of reaction products was acidic for all the systems due to formation of soluble acidic salts.
5. Optical and scanning micrographs indicated the presence of constituents in the reaction products as distinct phases.
References
1. C.R. Magana-Zavala, M.E. Angeles-San Martin, F.J. Rodriguez-Gomez, Anti-Corrosion Methods Mat. 56 (2009) 255. [ Links ]
2. P. Kofstad, G. Akkesson, Oxidation Metals 14 (1980) 301. [ Links ]
3. J.A. Goebel, F.S. Pettit, G.W. Goward, Metall. Mater. Trans. 4 (1973) 261. [ Links ]
4. J. Stringer, Annu. Rev. Mater. Sci. 7 (1977) 477. [ Links ]
5. R.A. Rapp, J.H. Devan, D.L. Dougass, P.C. Nordine, F.S. Petitt, D.P. Whittle, Mater. Sci. Eng. 50 (1981) 1. [ Links ]
6. D.Z. Shi, R.A. Rapp, J. Electrochem. Soc. 133 (1986) 849. [ Links ]
7. R.A. Rapp, Corrosion NACE 42 (1986) 568. [ Links ]
8. M. Mobin, A.U. Malik, J. Alloy Comp. 235 (1996) 97. [ Links ]
9. M. Mobin, A.U. Malik, S. Ahmad, S.K. Hasan, M. Ajmal, Bull. Mater. Sci. 19 (1996) 807. [ Links ]
10. M. Mobin, Sci. Eng. Comp. Mater. 8 (1999) 257. [ Links ]
11. JANAF Thermochemical Tables, NSRDS-NBS, Second ed., 1971. p.37.
12. O. Kubaschewski, E.L.L. Evans, C.B. Alcok, in Metallurgical Thermochemistry, Pergamon Press, London, 4th ed., 1967. [ Links ]
*Corresponding author. E-mail address drskhasan@yahoo.com
Received 25 January 2011; accepted 4 May 2011